Advancements in Accident Tolerance Fuel: A New Horizon in Nuclear Safety
DOI: http://dx.doi.org/10.55981/tdm.2024.7017
Abstract
Accident Tolerant Fuels (ATFs) represent a significant advancement in nuclear safety, offering the potential to mitigate the risks associated with nuclear reactor accidents. This paper provides a comprehensive overview of the development and current state of ATF technology, tracing its evolution and highlighting key technological milestones. Through an analysis of various case studies, we examine the practical application and performance of ATFs in real-world scenarios. Despite the promising capabilities of ATFs, their development and deployment are not without challenges. We delve into the technical, regulatory, and economic hurdles that must be overcome to realize the full potential of ATFs. Looking ahead, we explore the prospects of ATFs, discussing potential advancements and their implications for the nuclear industry. The findings of this paper underscore the transformative role of ATFs in enhancing nuclear reactor safety and charting a new horizon in nuclear technology.
Full Text:
PDFReferences
[1] Z. Karoutas et al., “The maturing of nuclear fuel: Past to Accident Tolerant Fuel,” Progress in Nuclear Energy, vol. 102, pp. 68–78, Jan. 2018, doi: 10.1016/j.pnucene.2017.07.016.
[2] Daniel S. Gomes, Rafael O. R. Muniz; Claudia Giovedi, “Improving Performance With Accident Tolerant-fuels,” in ASSOCIAÇÃO BRASILEIRA DE ENERGIA NUCLEAR – ABEN, Belo Horizonte: ASSOCIAÇÃO BRASILEIRA DE ENERGIA NUCLEAR – ABEN, Oct. 2017. [Online]. Available: https://inis.iaea.org/collection/NCLCollectionStore/_Public/48/103/48103601.pdf
[3] R. B. Rebak, Accident-tolerant materials for light water reactor fuels. Amsterdam, Netherlands ; Cambridge, MA, United States: Elsevier, 2020.
[4] D. Yun et al., “Current state and prospect on the development of advanced nuclear fuel system materials: A review,” Materials Reports: Energy, vol. 1, no. 1, p. 100007, Feb. 2021, doi: 10.1016/j.matre.2021.01.002.
[5] F. J. Goldner et al., “The U.S. Accident Tolerant Fuels Program — Update on a National Initiative,” Research Org.: Oak Ridge National Lab. (ORNL), Oak Ridge, TN (United States), Oct. 2021. [Online]. Available: https://www.osti.gov/biblio/1873835
[6] NEA-OECD, “State-of-the-Art Report on Light Water Reactor Accident-Tolerant Fuels,” NEA-OECD, 2018.
[7] K. E. Johnson et al., “Impact of fission product inclusion on phase development in U3Si2 fuel,” Journal of Nuclear Materials, vol. 537, p. 152235, Aug. 2020, doi: 10.1016/j.jnucmat.2020.152235.
[8] Z. Guo, R. Dailey, Y. Zhou, Z. Sun, J. Wang, and M. L. Corradini, “Effect of ATF Cr-coated-Zircaloy on BWR In-vessel Accident Progression during a Station Blackout,” Nuclear Engineering and Design, vol. 372, p. 110979, Feb. 2021, doi: 10.1016/j.nucengdes.2020.110979.
[9] K. K. Yadav, U. Pal, and K. R., “Concept of accident tolerant fuel in nuclear reactors,” Nuclear and Particle Physics Proceedings, vol. 341, pp. 62–66, Dec. 2023, doi: 10.1016/j.nuclphysbps.2023.09.026.
[10] B. Zhang, P. Gao, T. Xu, M. Gui, and J. Shan, “Performance evaluation of Accident Tolerant Fuel under station blackout accident in PWR nuclear power plant by improved ISAA code,” Nuclear Engineering and Technology, vol. 54, no. 7, pp. 2475–2490, Jul. 2022, doi: 10.1016/j.net.2022.01.024.
[11] M. Y. M. Mohsen, M. A. E. Abdel-Rahman, A. Omar, N. Alnassar, and A. A. Galahom, “Searching for the viability of using thorium-based accident-tolerant fuel for VVER-1200,” Nuclear Engineering and Technology, p. S1738573323004291, Sep. 2023, doi: 10.1016/j.net.2023.09.021.
[12] Gilles Youinou and R. Sonat Sen, “Enhanced Accident Tolerant Fuels for LWRS - A Preliminary Systems Analysis,” INL/EXT-13-30211, 1111499, Sep. 2013. doi: 10.2172/1111499.
[13] W. Li, K. Shirvan, S. Harrison, and J. Pegna, “Innovative accident tolerant fuel concept enabled through direct manufacturing technology,” Applied Energy, vol. 264, p. 114742, Apr. 2020, doi: 10.1016/j.apenergy.2020.114742.
[14] P. L. King, “Advanced Fuels Campaign Execution Plan,” United States, Jul. 2021. [Online]. Available: https://www.osti.gov/biblio/1813552
[15] L. Malerba et al., “Materials for Sustainable Nuclear Energy: A European Strategic Research and Innovation Agenda for All Reactor Generations,” Energies, vol. 15, no. 5, p. 1845, Mar. 2022, doi: 10.3390/en15051845.
[16] Lori Braase, “Enhanced Accident Tolerant LWR Fuels National Metrics Workshop Report,” INL/EXT-13-28090, 1073785, Jan. 2013. doi: 10.2172/1073785.
[17] S. J. Zinkle, “Opportunities and Challenges for Materials Innovation in Nuclear Energy,” EPJ Web of Conferences, vol. 51, p. 01001, 2013, doi: 10.1051/epjconf/20135101001.
[18] J. Strumpell, “Enhanced accident-tolerant fuel (EATF),” Nuclear Energy Agency of the OECD (NEA), Jun. 2013. [Online]. Available: http://inis.iaea.org/search/search.aspx?orig_q=RN:47013913
[19] J. Carmack, F. Goldner, S. M. Bragg-Sitton, and L. L. Snead, “Overview of the U.S. DOE Accident Tolerant Fuel Development Program,” in Report Number: INL/CON-13-29288, Research Org.: Idaho National Lab. (INL), Idaho Falls, ID (United States), Sep. 2013. [Online]. Available: https://www.osti.gov/biblio/1130553
[20] R. B. Rebak, “Innovative accident tolerant nuclear fuel materials will help extending the life of light water reactors,” Koroze a ochrana materialu, vol. 66, no. 1, pp. 36–39, Jan. 2022, doi: 10.2478/kom-2022-0006.
[21] S. M. Bragg-Sitton, M. Todosow, R. Montgomery, C. R. Stanek, R. Montgomery, and W. J. Carmack, “Metrics for the Technical Performance Evaluation of Light Water Reactor Accident-Tolerant Fuel,” Nuclear Technology, vol. 195, no. 2, pp. 111–123, Aug. 2016, doi: 10.13182/NT15-149.
[22] S. M. Bragg-Sitton, “Status Report on Activities of the Systems Assessment Task Force, OECD-NEA Expert Group on Accident Tolerant Fuels for LWRs,” INL/EXT--17-43389-Rev000, 1473588, Sep. 2017. doi: 10.2172/1473588.
[23] B. Gong et al., “UN and U3Si2 composites densified by spark plasma sintering for accident-tolerant fuels,” Ceramics International, vol. 48, no. 8, pp. 10762–10769, Apr. 2022, doi: 10.1016/j.ceramint.2021.12.292.
[24] X. Chen et al., “Investigations of the stability and electronic structures of U3Si2-Al: A first-principles study,” Chemical Physics, vol. 543, p. 111088, Mar. 2021, doi: 10.1016/j.chemphys.2020.111088.
[25] C. Moczygemba, J. George, E. Montoya, E. Kim, G. Robles, and E. Sooby, “Structure characterization and steam oxidation performance of U3Si2 with Zr alloying additions,” Journal of Nuclear Materials, vol. 570, p. 153951, Nov. 2022, doi: 10.1016/j.jnucmat.2022.153951.
[26] N. Mitsuboshi and H. Sagara, “Effects of U3Si2 fuel and minor actinide doping on fundamental neutronics, nuclear safety, and security of small and medium PWRs in comparison to conventional UO2 fuel,” Annals of Nuclear Energy, vol. 153, p. 108078, Apr. 2021, doi: 10.1016/j.anucene.2020.108078.
[27] K. Yang et al., “Cr-incorporated uranium nitride composite fuels with enhanced mechanical performance and oxidation resistance,” Journal of Nuclear Materials, vol. 559, p. 153486, Feb. 2022, doi: 10.1016/j.jnucmat.2021.153486.
[28] J. Yang et al., “Microstructure, mechanical properties and corrosion behavior of AlxCrMoNbZr multiprincipal element alloy coatings for accident-tolerant fuel claddings: Effect of Al content,” Surface and Coatings Technology, vol. 444, p. 128656, Aug. 2022, doi: 10.1016/j.surfcoat.2022.128656.
[29] N. M. George, J. J. Powers, G. I. Maldonado, K. A. Terrani, and A. Worrall, “Neutronic Analysis of Candidate Accident-tolerant Cladding Concepts in Light Water Reactors,” Research Org.: Oak Ridge National Lab. (ORNL), Oak Ridge, TN (United States), Jan. 2014. [Online]. Available: https://www.osti.gov/biblio/1185572
[30] P. J. Doyle et al., “Evaluation of the effects of neutron irradiation on first-generation corrosion mitigation coatings on SiC for accident-tolerant fuel cladding,” Journal of Nuclear Materials, vol. 536, p. 152203, Aug. 2020, doi: 10.1016/j.jnucmat.2020.152203.
[31] D. W. Kamerman, C. K. Jensen, N. E. Woolstenhulme, and D. M. Wachs, “Fuel Safety Research Plan for Accident Tolerant Fuels,” United States, Apr. 2019. [Online]. Available: https://www.osti.gov/biblio/1604971
[32] K. Nimishakavi and C. Lewis, “Development of LWR Fuels with Enhanced Accident Tolerance (EATF Phase 2 Final Scientific Technical Report),” FS1-0041211, 1545341, Dec. 2018. doi: 10.2172/1545341.
[33] US NRC, “Review of Accident Tolerant Fuel Concepts with Implications to Severe Accident Progression and Radiological Releases,” US NRC, ERI/NRC 20-209, Oct. 2020.
[34] Y. Che, G. Pastore, J. Hales, and K. Shirvan, “Modeling of Cr2O3-doped UO2 as a near-term accident tolerant fuel for LWRs using the BISON code,” Nuclear Engineering and Design, vol. 337, pp. 271–278, Oct. 2018, doi: 10.1016/j.nucengdes.2018.07.015.
[35] S. Kashibe and K. Une, “Effect of additives (Cr2O3, Al2O3, SiO2, MgO) on diffusional release of from UO2 fuels,” Journal of Nuclear Materials, vol. 254, no. 2–3, pp. 234–242, Apr. 1998, doi: 10.1016/S0022-3115(97)00356-5.
[36] S.-C. Jeon, D.-J. Kim, D. S. Kim, J.-H. Yang, and K.-S. Moon, “Thermal diffusion kinetics of cesium in ceramic microcell UO2 fuels for accident-tolerant fuel,” Journal of the European Ceramic Society, vol. 41, no. 13, pp. 6784–6788, Oct. 2021, doi: 10.1016/j.jeurceramsoc.2021.05.057.
[37] K. Li, L. Qian, X. Li, Y. Ma, and W. Zhou, “BeO Utilization in Reactors for the Improvement of Extreme Reactor Environments - A Review,” Front. Energy Res., vol. 9, p. 669832, May 2021, doi: 10.3389/fenrg.2021.669832.
[38] J. Song et al., “Theoretical investigations on the U2Mo3Si4 compound from first-principles calculations,” Progress in Nuclear Energy, vol. 118, p. 103121, Jan. 2020, doi: 10.1016/j.pnucene.2019.103121.
[39] I. W. Ngarayana et al., “Exploring the effects of Al and Si dopants on the accident tolerant fuels of UO2 pellets for light water reactor,” Nuclear Engineering and Design, vol. 418, p. 112902, Mar. 2024, doi: 10.1016/j.nucengdes.2024.112902.
[40] E. S. Wood et al., “High temperature steam oxidation dynamics of U3Si2 with alloying additions: Al, Cr, and Y,” Journal of Nuclear Materials, vol. 533, p. 152072, May 2020, doi: 10.1016/j.jnucmat.2020.152072.
[41] C. Yan et al., “High temperature steam oxidation performance of uranium dioxide based accident tolerant fuel,” Annals of Nuclear Energy, vol. 171, p. 109044, Jun. 2022, doi: 10.1016/j.anucene.2022.109044.
[42] A. García-Berrocal, C. Montalvo, and J. Blázquez, “Temperature transients in TRISO type fuel,” Annals of Nuclear Energy, vol. 76, pp. 172–176, Feb. 2015, doi: 10.1016/j.anucene.2014.09.031.
[43] N. R. Brown, “A review of in-pile fuel safety tests of TRISO fuel forms and future testing opportunities in non-HTGR applications,” Journal of Nuclear Materials, vol. 534, p. 152139, Jun. 2020, doi: 10.1016/j.jnucmat.2020.152139.
[44] J. Bischoff et al., “Development of Cr-coated Zirconium Alloy Cladding for Enhanced Accident Tolerance,” in Topfuel 2016 - Light Water Reactor (LWR) Fuel Performance Meeting, Boise, United States, Sep. 2016. [Online]. Available: https://hal-cea.archives-ouvertes.fr/cea-02438699
[45] H.-G. Kim, J.-H. Yang, W.-J. Kim, and Y.-H. Koo, “Development Status of Accident-tolerant Fuel for Light Water Reactors in Korea,” Nuclear Engineering and Technology, vol. 48, no. 1, pp. 1–15, Feb. 2016, doi: 10.1016/j.net.2015.11.011.
[46] Y. Che, X. Wu, G. Pastore, W. Li, and K. Shirvan, “Application of Kriging and Variational Bayesian Monte Carlo method for improved prediction of doped UO2 fission gas release,” Annals of Nuclear Energy, vol. 153, p. 108046, Apr. 2021, doi: 10.1016/j.anucene.2020.108046.
[47] W. Zhou and W. Zhou, “Multiphysics Modeling of Fabrication Methods’ Effect on UO2-BeO Composite Fuels’ Performance,” in Volume 9: Student Paper Competition, London, England: American Society of Mechanical Engineers, Jul. 2018, p. V009T16A026. doi: 10.1115/ICONE26-81429.
[48] W. Zhou and W. Zhou, “Enhanced thermal conductivity accident tolerant fuels for improved reactor safety – A comprehensive review,” Annals of Nuclear Energy, vol. 119, pp. 66–86, Sep. 2018, doi: 10.1016/j.anucene.2018.04.040.
[49] E. Alat, M. J. Brova, I. M. Younker, A. T. Motta, M. Fratoni, and D. E. Wolfe, “Neutronic and mechanical evaluation of rare earth doped and undoped nitride-based coatings for accident tolerant fuels,” Journal of Nuclear Materials, vol. 518, pp. 419–430, May 2019, doi: 10.1016/j.jnucmat.2019.02.044.
[50] B. Gong et al., “Cr-doped U3Si2 composite fuels under steam corrosion,” Corrosion Science, vol. 177, p. 109001, Dec. 2020, doi: 10.1016/j.corsci.2020.109001.
[51] P. Kegler et al., “Chromium Doped UO2-Based Ceramics: Synthesis and Characterization of Model Materials for Modern Nuclear Fuels,” Materials, vol. 14, no. 20, p. 6160, Oct. 2021, doi: 10.3390/ma14206160.
[52] Y. Zhong et al., “Preparation and Characterization of Large Grain UO2 for Accident Tolerant Fuel,” Front. Mater., vol. 8, p. 651074, Apr. 2021, doi: 10.3389/fmats.2021.651074.
[53] Q. Sun, Y. Yang, M. Li, P. Ji, and W. Liu, “Development Background and Research Progress of UN-U3Si2 Composite Fuel,” in Proceedings of the 23rd Pacific Basin Nuclear Conference, Volume 3, vol. 285, C. Liu, Ed., in Springer Proceedings in Physics, vol. 285. , Singapore: Springer Nature Singapore, 2023, pp. 715–723. doi: 10.1007/978-981-19-8899-8_69.
[54] Z. Li et al., “Review on performance of chromium-coated zirconium and its failure mechanisms,” Front. Nucl. Eng., vol. 2, p. 1212351, Jun. 2023, doi: 10.3389/fnuen.2023.1212351.
[55] J.-S. Jiang, D.-Q. Wang, M.-Y. Du, X.-F. Ma, C.-X. Wang, and X.-J. He, “Interdiffusion behavior between Cr and Zr and its effect on the microcracking behavior in the Cr-coated Zr-4 alloy,” NUCL SCI TECH, vol. 32, no. 12, p. 134, Dec. 2021, doi: 10.1007/s41365-021-00977-w.
[56] J. Ribis, A. Wu, J.-C. Brachet, F. Barcelo, and B. Arnal, “Atomic-scale interface structure of a Cr-coated Zircaloy-4 material,” J Mater Sci, vol. 53, no. 14, pp. 9879–9895, Jul. 2018, doi: 10.1007/s10853-018-2333-1.
[57] M. Wagih, B. Spencer, J. Hales, and K. Shirvan, “Fuel performance of chromium-coated zirconium alloy and silicon carbide accident tolerant fuel claddings,” Annals of Nuclear Energy, vol. 120, pp. 304–318, Oct. 2018, doi: 10.1016/j.anucene.2018.06.001.
[58] K. A. Unocic, Y. Yamamoto, and B. A. Pint, “Effect of Al and Cr Content on Air and Steam Oxidation of FeCrAl Alloys and Commercial APMT Alloy,” Oxid Met, vol. 87, no. 3–4, pp. 431–441, Apr. 2017, doi: 10.1007/s11085-017-9745-1.
[59] B. A. Pint, K. A. Terrani, Y. Yamamoto, and L. L. Snead, “Material Selection for Accident Tolerant Fuel Cladding,” Metallurgical and Materials Transactions E, vol. 2, no. 3, pp. 190–196, Sep. 2015, doi: 10.1007/s40553-015-0056-7.
[60] C. Schneider et al., “Microstructural Analysis of Zirconia at the Fuel-Cladding Interface in Medium and High Burnup Irradiated Fuel Rods,” Oxid Met, vol. 96, no. 3–4, pp. 295–306, Oct. 2021, doi: 10.1007/s11085-021-10045-8.
[61] A. T. Motta, A. Couet, and R. J. Comstock, “Corrosion of Zirconium Alloys Used for Nuclear Fuel Cladding,” Annu. Rev. Mater. Res., vol. 45, no. 1, pp. 311–343, Jul. 2015, doi: 10.1146/annurev-matsci-070214-020951.
[62] J. Bischoff et al., “PROtect: Accelerated Implementation of Framatome’s Evolutionary eATF Solution,” presented at the Light Water Reactor Fuel Performance Conference, Red Hook, NY, Seattle, Wash.: Curran Associates, Inc.;, 2020, pp. 109–114. [Online]. Available: https://www.tib.eu/de/suchen/id/TIBKAT%3A181003163X
[63] R. E. Stachowski, R. B. Rebak, W. P. Gassmann, and J. Williams, “Progress of GE development of accident tolerant fuel FeCrAl cladding,” Research Org.: American Nuclear Society - ANS, 555 North Kensington Avenue, La Grange Park, IL 60526 (United States): American Nuclear Society - ANS; La Grange Park, IL (United States), Jul. 2016. [Online]. Available: https://www.osti.gov/biblio/22750104
[64] L. Y.P. et al., “Path Towards Industrialization of Enhanced Accident Tolerant Fuel,” in TopFuel 2018, Prague Czech Republic, Oct. 2018.
[65] E. Lahoda, S. Ray, P. Xu, and F. Boylan, “Westinghouse Accident Tolerant Fuel Program,” Transactions of the American Nuclear Society, vol. 114, Jun. 2016, [Online]. Available: https://www.osti.gov/biblio/22992054
[66] X. P., L. E.J., L. J., D. C.P., and K. G.E., “STATUS UPDATE ON WESTINGHOUSE SiC COMPOSITE CLADDING FUEL DEVELOPMENT,” in TopFuel 2018, Prague Czech Republic, Oct. 2018.
[67] D. C.P. et al., “DEMONSTRATION OF ENGINEERED MULTI-LAYERED SIC-SIC CLADDING WITH ENHANCED ACCIDENT TOLERANCE,” in TopFuel 2018, Prague Czech Republic, Oct. 2018.
[68] F. Andrea, “Development and performance evaluation of accident-tolerant coated fuel claddings for light water reactors,” PhD Thesis, Chalmers University of Technology, Göteborg Sweden, 2023. [Online]. Available: https://research.chalmers.se/publication/533922/file/533922_Fulltext.pdf
[69] C. Parisi, Z. Ma, D. Mandelli, N. Anderson, and H. Zhang, “Risk-Informed Safety Analysis for Accident Tolerant Fuels,” Nuclear Science and Engineering, vol. 194, no. 8–9, pp. 748–770, Sep. 2020, doi: 10.1080/00295639.2020.1732699.
[70] Safety Analysis and Licensing Documentation for Nuclear Fuel Cycle Facilities. in Safety Reports Series, no. 102. Vienna: INTERNATIONAL ATOMIC ENERGY AGENCY, 2020. [Online]. Available: https://www.iaea.org/publications/13456/safety-analysis-and-licensing-documentation-for-nuclear-fuel-cycle-facilities
[71] D. T., S. J., V. C., and C. P., “Fuel Qualification for Advanced Reactors.” USNRC, Mar. 2022. [Online]. Available: https://www.nrc.gov/docs/ML2206/ML22063A131.pdf
[72] A. G. Sowder, “Challenges and Opportunities for Commercialization of Enhanced Accident Tolerant Fuel or Light Water Reactors: A Utility-Informed Perspective,” International Atomic Energy Agency (IAEA), 1011–4289, 2016. [Online]. Available: http://inis.iaea.org/search/search.aspx?orig_q=RN:47080124
[73] E. V. Semenov and V. V. Kharitonov, “Effects of Accident-Tolerant Nuclear Fuel on NPP Economic Parameters,” At Energy, vol. 133, no. 5–6, pp. 331–338, Mar. 2023, doi: 10.1007/s10512-023-01017-z.
[74] E. S. Wood, J. T. White, and A. T. Nelson, “The effect of aluminum additions on the oxidation resistance of U3Si2,” Journal of Nuclear Materials, vol. 489, pp. 84–90, Jun. 2017, doi: 10.1016/j.jnucmat.2017.02.045.
[75] A. Migdisov et al., “Instability of U3Si2 in pressurized water media at elevated temperatures,” Commun Chem, vol. 4, no. 1, p. 65, May 2021, doi: 10.1038/s42004-021-00504-3.
[76] B. Gong, T. Yao, P. Lei, J. Harp, A. T. Nelson, and J. Lian, “Spark plasma sintering (SPS) densified U3Si2 pellets: Microstructure control and enhanced mechanical and oxidation properties,” Journal of Alloys and Compounds, vol. 825, p. 154022, Jun. 2020, doi: 10.1016/j.jallcom.2020.154022.
[77] K. Fukuda and K. Iwamoto, “Concentration profiles of fission products in the coating layers of irradiated fuel particles,” Journal of Nuclear Materials, vol. 66, no. 1–2, pp. 55–64, 1977, doi: 10.1016/0022-3115(77)90133-7.
[78] P. A. Demkowicz, B. Liu, and J. D. Hunn, “Coated particle fuel: Historical perspectives and current progress,” Journal of Nuclear Materials, vol. 515, pp. 434–450, 2019, doi: 10.1016/j.jnucmat.2018.09.044.
[79] K. A. Terrani, “Accident tolerant fuel cladding development: Promise, status, and challenges,” Journal of Nuclear Materials, vol. 501, pp. 13–30, Apr. 2018, doi: 10.1016/j.jnucmat.2017.12.043.
[80] S. Bragg-Sitton et al., “Enhanced Accident Tolerant LWR Fuels: Metrics Development,” in Report Number: INL/CON-13-28394, Research Org.: Idaho National Lab. (INL), Idaho Falls, ID (United States), Sep. 2013. [Online]. Available: https://www.osti.gov/biblio/1122125
Refbacks
- There are currently no refbacks.
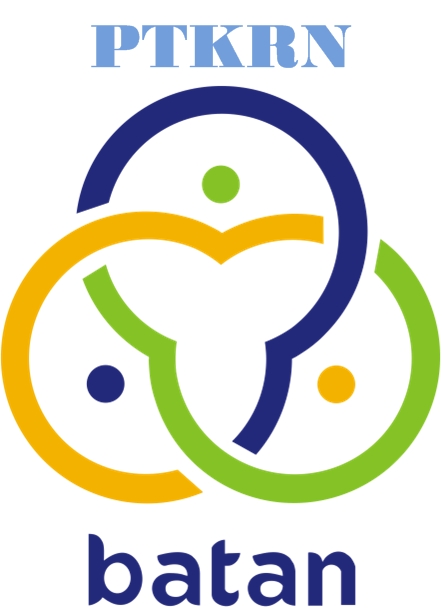
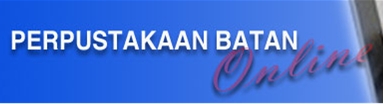
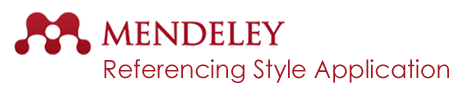